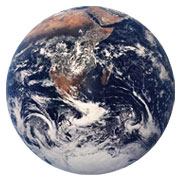
Keeping the lights on in the global industrial world — never an easy task — never seems to get any easier.
Nuclear energy, which provides nearly 20 percent of our nation’s electricity, is at a crossroads. Can nuclear reactors — the torrid, pulsating, heat-generating hearts of nuclear power plants — ever be safe enough?
Particularly following the May 2011 Fukushima Daiichi nuclear plant disaster in northern Japan, in which the cores of three of the plant’s six reactors melted down and still remain critically dangerous, there’s no doubt that everyone on this planet needs to know the answer to that question.

80 Years in Operation
All 103 nuclear reactors currently operational in the United States have irreparable safety issues and should be taken out of commission and replaced, according to Gregory Jaczko, former chairman of the U.S. Nuclear Regulatory Commission.
Many American reactors that had received permission from the NRC to operate for 20 years beyond their initial 40-year licenses probably would not last that long Jaczko said at the April 2013 Carnegie International Nuclear Policy Conference in Washington, D.C.
Changes proposed by the commission that would allow reactor owners to apply for a second 20-year extension — meaning that some reactors would run for a total of 80 years — also were unacceptable, he said.
The US$33 billion nuclear power industry officially disagrees with Jaczko, of course, insisting that all U.S. nuclear power plants are operating safely.
Full Steam Ahead
As they defend the present, though, the industry — and its sponsor, the U.S. Dept. of Energy — are plotting a vibrant “technonuke” future with a new generation of nuclear reactors. In essence, they’re promoting the possibility of cheap, clean, safe and small-scale nuclear power as essential for our energy-hungry way of life.
In Jan. 2012, the NRC certified the Westinghouse Electric AP1000 nuclear reactor, the design chosen for power plants proposed for construction across the U.S. Southeast by Duke Energy, Southern Company, Florida Power & Light, Progress Energy, Tennessee Valley Authority and SCANA.
The AP1000 was supported through a cost-shared agreement with the DOE.
The AP1000 is being built at two sites in Georgia and South Carolina — and at two sites in China. The design incorporates safety features that rely on natural forces like gravity and natural circulation to ensure nuclear core cooling is maintained in the event of a loss of normal cooling, according to Steve Kerekes, a spokesman for the Nuclear Energy Instituteindustry lobbying group.
“Facilities with these nonmechanical features include the safety advancements common to all new reactor designs, plus the additional benefits of even greater safety margins, safety assured by natural forces — e.g., gravity — natural circulation, no reliance on AC power and reduced reliance on operator action to respond to emergencies,” Kerekes told TechNewsWorld.
A ‘Traveling Wave’ Reactor
Even private citizen investors like billionaire Bill Gates are getting into the fission game.
Gates backs TerraPower, a Bellevue, Wash., a nuclear reactor design startup. The company’s TWR-P is a 600 MWe demonstration “traveling wave” reactor designed to convert fertile material into fissile fuel as it operates.
MWe means “megawatt electrical,” a term that refers specifically to electric power; a megawatt means there’s enough juice available to simultaneously light up one million one-watt or 10,000 100-watt light bulbs.
In any case, the TWR-P’s purported economic benefits stem from its ability to “breed” and burn metallic fuel comprised of an initial starter fuel of U-235 and U-238 isotopes. The “traveling-wave” name refers to the fact that fission does not occur throughout the entire TWR-P core, but remains confined to a boundary zone that slowly advances through the core over time.
1974 or Earlier
Though nuclear reactors are used in other applications as well, such as submarine propulsion and laboratory research, most Americans know them as the business end of a nuclear power plant.
The U.S. has 103 nuclear power reactors at 65 plants in 31 states, operated by 30 different power companies, according to the World Nuclear Association.
Of the U.S. reactors still operational, ground was broken on all of them in 1974 or earlier. There has been no new groundbreaking on nuclear plants in the U.S. since 1974, though a number of reactor units started before 1974 have been completed since then.
More recently, though — in 2011 and 2012 — construction has begun on new units at existing plants. There are now five such plants under construction in the United States: Watts Bar 2 in Tennessee, Summer 2 and Summer 3 in South Carolina, and Vogtle 3 and Vogtle 4 in Georgia.
The core of a nuclear reactor contains both water and assemblies of fuel rods clad in zirconium and containing ceramic pellets of U-235-enriched nuclear fuel. This fuel is bombarded with neutrons to set off controlled nuclear reactions. These reactions super-heat the water, creating a stream that measures 550 degrees Fahrenheit and powers a turbine, generating electricity.
Light Water Reactors
Most operating nuclear power plants use thermal reactors — the most common types of which are light water reactors. These use normal water instead of “heavy water”as both coolant and neutron moderator.
For fuel, LWRs use a solid compound of fissile elements. There are three varieties of LWRs: boiling water reactor, pressurized water reactor and (most designs of) the supercritical water reactor.
The Fukushima Daiichi reactors are boiling water reactors of an early (1960s) design supplied by GE, Toshiba and Hitachi. Reactors 1, 2 and 3 — which experienced full meltdown — came into commercial operation between 1971 and 75.
PWRs, on the other hand, constitute the large majority of all Western nuclear power plants. In a PWR, water — the primary coolant — is pumped under high pressure to the reactor core, where it is heated by the energy generated by atomic fission.
Five Generations
Nuclear reactors are classified by type of nuclear reaction, by moderator material, by coolant and, most commonly, by generation:
- Gen I are the power reactors that launched civil nuclear power in the 1950s.
- Gen II reactors, including PWRs and BWRs, were installed between 1965 and 1996 and are currently in use at most of the world’s 400+ nuclear power plants.
- Gen III reactors, which incorporate evolutionary improvements on existing Gen II designs, have been implemented between 1996 and now. Improvements are in the areas of fuel technology, thermal efficiency, modularized construction, safety systems (especially the use of passive rather than active systems) and standardized design.
- Gen IV reactor technologies are years in the future. They include concepts such as gas-cooled fast reactors, lead-cooled fast reactors, liquid metal-cooled reactors, molten salt reactors, sodium-cooled fast reactors, supercritical water-cooled reactors and very high-temperature gas reactors.
- Gen V are exotics like plasma fusion reactors, in which two light atomic nuclei fuse together to form a heavier nucleus — in contrast to the opposite reaction in fission power. The liquid fluoride thorium reactor is a thermal breeder reactor that uses the thorium fuel cycle in a fluoride-based molten (liquid) salt fuel to achieve high operating temperatures at atmospheric pressure.
Small Modular Reactors
One joint government-industry strategy to revitalize Big Nuke is the Small Modular Reactor — smaller supposedly means less expensive and more efficient.
SMRs are part of a new generation of nuclear power plant designs being developed in several countries. The objective of these SMRs is to provide a flexible, cost-effective energy alternative.
While standard nuclear plant reactors put out 1,100 or more MWe, SMRs are defined by the International Atomic Energy Agency as those with an electricity output of less than 300 MWe, although anything with an output of less than 500 MWe is widely considered to count as a small reactor.
SMRs could be made in factories and transported to sites where they would be ready to “plug and play” upon arrival, in theory reducing both capital costs and construction times. The small size also is intended to make Small Modular Reactors suitable for small electric grids and for locations that cannot support large reactors, providing utilities with the flexibility to scale production as demand changes.
The smaller, simpler design of modular reactors makes it possible for a power company to scatter them in more sites — maybe even a decommissioned coal plant — or to cluster them to get the effect of a full-sized reactor, proponents say.
Of course, that also means more sites where nuclear materials will have to be stored or where accidents or terrorist attacks could occur, detractors say.
Leading SMR companies include the Westinghouse/Ameren consortium (225MWe Westinghouse SMR), Bechtel/B&W (mPower reactor), Fluor/NuScale Power (45MW NuScale design) and Holtec International (SMR-160).
On Jan. 20, 2012, the DOE announced the first step in a US$452 million program toward manufacturing SMRs in the United States. The full grant award will fund up to two SMR designs, with the goal of deploying these reactors by 2022.
A New Facility

On November 20, 2012, the DOE announced that the Babcock & Wilcox Company and its “mPower” reactor were the winners of its Funding Opportunity Announcement grant award for a cost-sharing program with industry for the design and licensing of SMRs.
B&W leads the project, supported by the award in partnership with the Tennessee Valley Authority and Bechtel Corp. If TVA’s partnership with B&W goes as planned, B&W and Bechtel will build a reactor and TVA will build a facility for it at TVA’s Clinch River site in Oak Ridge.
The flexibility and scalability of SMRs provides an important alternative for the development of nuclear power generation, said Greg Ashley, president of Bechtel’snuclear business line.
“Compared to traditional large reactor nuclear technologies, SMR technology offers enhanced safety and security, lower initial capital cost, shorter construction schedule as a result of the use of factory-built modules, and competitive generation costs,” Ashley told TechNewsWorld.
Underwater and Underground
Also in the running for a DOE grant is NuScale Power, based in Corvallis, Ore. NuScaleis the only U.S.-based company established solely for the deployment and commercialization of SMR technology.

Using LWR technology, the NuScale Power Module is cooled by natural circulation. The reactor is entirely self-contained and installed underwater and underground for safety purposes, the company says.
At 45 MWe each, the company’s NuScale Power Module is “the only truly small SMR,” it says.
The NPM consists of an integral reactor vessel, containment vessel, steam generator and pressurizer, all in a single cylindrical vessel that can be factory-built and transported to the installation site, according to Mike McGough, NuScale’s chief commercial officer.
“NPMs can be installed in groups of as many as 12 to comprise a 540 MWe installation,” McGough told TechNewsWorld. “The NPMs are all installed in individual underground operating bays, immersed in an 11-million-gallon common pool of water.”
The NuScale design is the only design of any kind that can safely shut down and cool indefinitely with no operator action, no power and no additional water, he added. “This is a revolutionary triple crown of nuclear plant safety.”
Not So Fast
Not surprisingly, SMRs still have their critics.
“The Union of Concerned Scientists has long been concerned about the safety and security risks of SMRs, especially if the vendors and DOE succeed in convincing the NRC to weaken regulatory requirements in areas such as plant staffing, security and emergency planning zone size,” warned Ed Lyman, UCS senior scientist, in a 2012 report entitled, “Does DOE’s Funding Announcement Mark the End of its Irrational Exuberance for SMRs?”
“We articulated these concerns in our testimony to the Senate in July 2011, and in testimony to the Secretary of Energy Advisory Board in May 2012,” Lyman said, “but have not seen any indication that our concerns are being seriously addressed.”
HA!
I thought i was the only one that knew about Thorium
Also
India is building a Thorium reactor
Of all those technologies, I (and a fast growing number of people) feel that Thorium Molten Salt Reactors (also known as Liquid Fluoride Thorium Reactors or LFTR) hold the most promise.
The key is in the fact the fuel is a liquid rather than a solid – solid fuels inherently can’t "circulate". As a consequence they achieve less than 1% burnup rate. They also crack and deform due to fission products such as the gas Xenon-135. As such, the fuel rods must be removed and recycled on a regular basis (or in the US, treated as waste due to a draconian non-proliferation ban on reprocessing of spent fuel), during which time the reactor has to be taken offline.
With a Molten Salt Reactor on the other hand, you can achieve a 99% fuel burnup rate, leaving you with a vastly reduced quantity of waste. The fission products can also be removed with the reactor staying on-line, meaning MSRs can have a capacity factor of above 95%.
If you lose containment, the fuel leaks out and solidifies into a lump of salt, easily scooped up and put back in the reactor. There is also an exceptional safety feature – a passively cooled tank is situated below the main reactor vessel, connected by a pipe, with a fan blowing over it. The fan cools and freezes a lump of salt. If the reactor building loses power, the plug melts and the fuel drains into the drain tank, leaving the reactor in a completely safe walk-away state. This is an astonishing level of safety.
Because MSRs can use Thorium, they have the potential to produce energy cheaper than coal – Thorium is as abundant as lead and found everywhere on Earth. It’s a byproduct of the mining industry, people will pay you to take it away. There is enough of the stuff to supply our energy needs until past the death of our star. We will literally never run out.
There’s an excellent video on Youtube called "Thorium Remix 2011" I’d recommend watching which explains the technology in great detail. There’s also a documentary being made in Ireland called "The Good Reactor" that’s on Kickstarter at the moment, which is worth looking up.
Lastly, this isn’t a future theoretical technology, Oak Ridge National Labs designed and built one in the 60s, and operated it for 5 years. China is building one at its National Academy of Sciences, as is a joint collaboration between Japan and the Czech Republic called the Fuji Molten Salt Reactor.
Molten Salt Reactors have the potential to be a *game changer*. Right now nothing can challenge coal for price per kWh. This can. That will change everything.